What is Controlled Impedance?
Unless you have carefully designed the trace and its environment, impedance is typically “uncontrolled”, meaning that impedance will vary in value from point to point along the trace.
At high frequencies, PCB traces do not behave like simple connections, controlled impedance helps us ensure that signals are not degraded as they route around a PCB.
Essentially, controlled impedance is the matching of substrate material properties with trace dimensions and locations to ensure the impedance of a trace’s signal is within a certain percentage of a specific value. Controlled impedance boards provide repeatable high frequency performance.
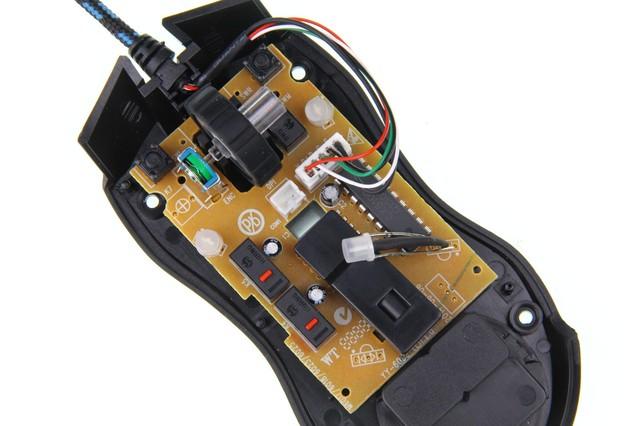
Why is impedance matching needed?
The function of a PCB trace is to transfer the signal power from the driver device to the receiving device.
Power needs to be propagated throughout the length of the trace. But maximum signal power can only be achieved with matching impedances on the PCB.
So, that is why there is a need for impedance matching. We want as much of the power from the driver to end up at the receiver.
If special care is not taken in the PCB layout stage, then high-frequency signals will definitely degrade as they propagate from the driver to the receiver. If the result of this is viewed on an eye diagram the signals will be very distorted and power levels will be different as the signal propagates from the start to the end.

How do I make controlled impedance traces on my PCB?
Traces with a characteristic impedance come in roughly four flavours:
- a single conductor over a reference plane (“microstrip”)
- a single conductor between two reference planes (“stripline”)
- two conductors over a reference plane (“differential microstrip”)
- two conductors between two reference planes (“differential stripline”)
For each configuration, you can find formulas and calculators online that do the calculations for you. The calculators are available as simple websites where one enters the data, up to high end field solver software that considers all environmental parameters in the calculations. I find Saturn PCB’s PCB Toolkit a very practical and feature-rich tool.
The impedance of a single trace over a reference plane is determined by:
- the width of the trace (a wider trace has a lower impedance)
- the distance between the trace and the reference plane (a smaller distance yields a lower impedance)
- the relative dielectric constant εr of the PCB material (a higher εr yields a lower impedance)
- to a lesser extent the copper thickness (thicker copper yields slightly lower impedances)
Differential conductors can be regarded as two single traces that have a lower mutual impedance as they are brought closer together. The calculation of a single impedance controlled trace will have one solution, whereas differential traces offer some room for tinkering with the trace width and spacing.
Here are some tips:
Find out what PCB layer stackup and materials your PCB manufacturer
will use to fabricate your PCB. These are the most important parameters for controlled impedance traces.
traces.
- FR-4 does not have a very constant εr over frequency, so find the εr that matches the
frequency of your application.
Choose a PCB material that is suited for RF whenever the application requires high frequencies (>1 GHz),
high power (>1 Watt) and long distances over the PCB (> 10 cm).
Don’t make the traces very thin. Variations in the etching process lead to variations in trace
width and thus in trace impedance. I like to maintain a minimum trace width of 200 µm.
Maintain a clearance around controlled impedance traces to reduce interaction with
the surrounding copper. A rule of thumb is 5…7 times the
trace width.
Don’t use bends sharper than 45° in controlled impedance traces.
To also provide some practical examples, I’ve calculated some trace widths on the outer layers of Eurocircuits’ standard 4-layer FR-4 PCBs:
- Z0 = 50 Ohm (antennas, cables): 650 µm track width
- Z0 = 75 Ohm (antennas, cables): 280 µm track width
- ZDIFF = 90 Ohm (USB): 500 µm track width with 300 µm spacing or 400 µm
track width with 150 µm spacing.
- ZDIFF = 100 Ohm (USB): 350 µm track width with 200 µm
spacing or 300 µm track width with 150 µm spacing.

Applications of Controlled Impedance
Controlled Impedance should be considered for PCBs used in fast digital applications such as:
